Introduction
Tinnitus, perceiving sound in the brain or ear without an external sound source, has affected the quality of life for millions of people worldwide. The prevalence of this phantom feeling is estimated at 10%-15% [
1], although up to 30% has been reported [
2]. Although the prevalence of tinnitus increases with age, chronic tinnitus can occur at any age [
3], even in children [
4]. Among people with tinnitus, 1%-3% suffer from emotional distress, cognitive dysfunction, and or autonomic arousal caused by tinnitus [
5], and the term “tinnitus disorder” has recently been introduced to describe this type of tinnitus [
6].
No valid treatment for chronic tinnitus can eliminate the symptoms [
7, 8]. Differences in the definition of tinnitus, heterogeneity, and problems measuring tinnitus challenge the tinnitus studies [
9]. Consequently, the exact pathophysiological pathways are still not fully understood [
10]. Various networks seem to be responsible for diverse aspects of tinnitus. Based on the integrative or global workspace model, the degree of involvement of loudness, memory, awareness, salience, and distress subnetworks causes tinnitus subtypes and variability in different people [
11].
The comprehensive, objective assessment methods facilitate the investigation of neurophysiological hypotheses that lead to efficacious therapeutic solutions. Correspondingly, it became possible to subphenotype tinnitus, which is the basis for standardizing tinnitus assessment methods.
Objective tinnitus testing overcomes the limitations of subjective assessment, such as individual and intra-individual variability, non-usability in certain populations, and pediatric and forensic medicine [
12]. It is also likely to help detect tinnitus in an earlier phase. In chronic tinnitus with symptoms lasting more than 3 months, tinnitus-related networks may become progressively centralized and resistant to treatment [
13, 14].
To date, the clinical diagnosis of tinnitus in humans has only been based on subjective methods, such as self-assessment questionnaires, visual rating scales, and psychoacoustic assessments. Various objective methods have been employed to investigate tinnitus in humans, such as electroencephalography (EEG), magnetoencephalography, auditory brainstem responses (ABR), cortical auditory evoked potentials (CAEP), auditory evoked magnetic field, functional magnetic resonance imaging, positron emission tomography, single photon emission computed tomography, molecular genetics and blood-based biochemical markers [
15]. CAEP can potentially be employed as a non-invasive objective tool in tinnitus measurement. CAEP offers a good temporal and reasonable spatial resolution, such as high-density auditory evoked potentials (AEPs). Tinnitus has distinct components, including perceptual characteristics (such as pitch, loudness, timbre, and location) and its effects on behavior and emotion. Each current tool (psychoacoustic assessments and questionnaires) measures only one aspect of perception or impact, and it seems reasonable and necessary to estimate the different aspects of tinnitus in light of the workspace model. The source localization of multi-channel CAEPs provides a comprehensive assessment and holistic comprehension of tinnitus by spatial examination of different auditory and non-auditory neural hubs and networks. Another advantage of CAEPs over other objective methods is using specific stimulus protocols to investigate the probable central mechanisms of tinnitus. For instance, comparing CAEP parameters at frequencies with and without hearing loss can be used to study discordant frequency-dependent map reorganization in the auditory cortex in line with edge theory [
16, 17]. Another special stimulus paradigm considered in this study is the gap pre-pulse inhibition of acoustic startle (GPIAS). This protocol can examine gap processing and temporal acuity by the auditory cortex.
GPIAS is one of the modifications of acoustic startle reflex (ASR), the most widely used method to detect tinnitus in animals, and is currently being studied in humans. The startle reflex involves the contraction of the facial and skeletal muscles and autonomic physiological response that reacts to strong and sudden stimuli in various modalities (visual, auditory, tactile) [
18]. It can be recorded in different species and holds several crucial types of plasticity that are good candidates for research on brain mechanisms. These startle reflex modulations include pre-pulse inhibition (PPI), gap pre-pulse inhibition (GPI), habituation, sensitization, and fear potentiation.
The results of animal studies implicate that the cortico-striatal-pallido-thalamic circuit generates PPI. The neural circuitry of PPI in rodents reflects automated processing in the pre-attention phase. Still, it can be modulated by cognitive processing due to the descending axons it receives from the forebrain [
19]. Although GPIAS is a kind of PPI, they are probably different regarding temporal features and neural circuits involved in gap detection. The auditory cortex seems to be one of the essential areas for GPIAS but it is not for PPI [
20, 21]. Although the PPI circuit is well established in animals and many similarities exist between the measurements of the PPI between rodents and humans [
22], the neural pathways of the PPI in humans are poorly understood and are still hypothesized. Human behavioral, physiological, and pharmacological studies suggest a complex neural network from the brainstem to higher-level cortical regions and the connections between the thalamus and striatum with the temporal, prefrontal, frontal, and parietal cortices [
23, 24, 25]. Common neural areas exist in the PPI/GPIAS and tinnitus network. The extensive anatomical overlap between the PPI/GPIAS regulatory regions and the tinnitus networks supports that tinnitus, as a deficit of sensory-gating disorder, can affect the GPIAS and that cortical recordings can trace these effects. Thus, the present study aims to investigate in the literature whether CAEP with the GPIAS paradigm can be utilized as an objective tool to identify or classify tinnitus in humans according to the same acoustic sensory input pathway of CAEP and GPIAS.
Materials and Methods
Studies were identified by searching electronic databases and trial registers of PubMed, EMBASE, Medline, ClinicalTrials.gov, Google Scholar, and Web of Science. Keywords and equivalent terms applied alone or in combination were tinnitus, ear ringing, or phantom auditory sensation; acoustic startle reflex, pre-pulse inhibition, gap pre-pulse inhibition acoustic startle, ASR, PPI, or GPIAS; objective tinnitus assessment, objective tinnitus evaluation, objective tinnitus measurement, tinnitus detection with stimuli included gap, gap-induced auditory evoked potentials, tinnitus-related neural activity, gap detection, temporal resolution or temporal acuity; EEG, auditory evoked potentials (AEP), CAEP, auditory late latency response (ALLR), auditory middle latency response (AMLR), ABR, auditory cortex or A1. Our search was limited to available peer-reviewed and full-text journal articles written in English from 2006 onwards that directly addressed the gap-elicited assessments in humans with tinnitus.
Results
By searching for the keywords mentioned above, 93 related articles were obtained, of which 73 studies were conducted on animals and 20 on humans. The GPIAS method was performed in animal studies on different species (mice, guinea pigs, hamsters, gerbils, and rats) after inducting tinnitus by pharmacological methods (salicylate and quinine) or exposure to loud noises [
26]. Turner first introduced the GPIAS method for rapid tinnitus screening in animal models. Subsequently, GPIAS was used in several studies to measure tinnitus in animal models. The stimuli used for GPIAS in animal studies included broadband noise (BBN) or narrowband noise (NNB) with a central frequency ranging from 500 Hz to 36 kHz and a presentation intensity of 55 to 120 dB sound pressure level (SPL). The gap in the stimulus differed in durations from 2 to 100 ms. Most studies have considered a statistically significant decrease in GPIAS compared to the control group as a measure of tinnitus presence. Some studies devoted a statistical comparison of the startle amplitude in the trials with and without gaps to confirm the existence of tinnitus. If the amplitude was not significant in these two conditions, it indicated the presence of tinnitus in the animal. Another method was to consider a fixed threshold so the tinnitus group would be regarded as if the GPIAS ratio were above that threshold. The control group would be considered if this ratio was below the threshold.
In 20 human studies of search, three silent gap-based stimulus patterns were used, including the gap in noise (GIN), multi-deviant mismatch negativity (MMN), and GPIAS paradigms. Nine out of 20 studies examined these stimulus patterns in normal individuals without tinnitus, which were not considered in this study.
Table 1 summarizes the characteristics of the other 11 studies performed in the tinnitus group.
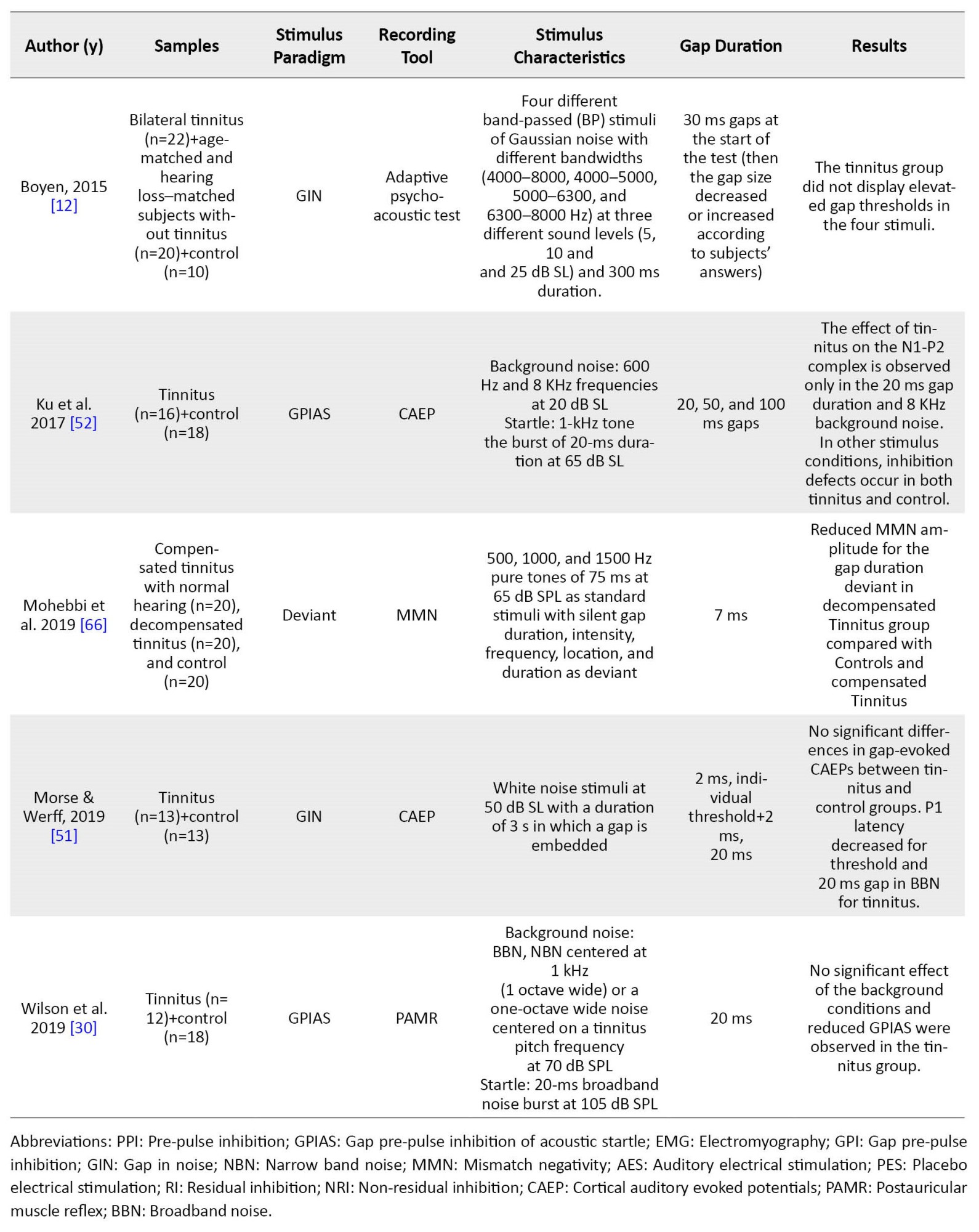
Several ways were found to examine the GPIAS paradigm and discover the gap in human studies. Some studies employed direct behavioral measurements of gap detection and psychometric functions. In this regard, two psychoacoustic studies analyzed the conscious detection of silent gaps [
12,
27]. Because the acoustic startle response involves many muscles, including limbs and head muscles, components of the startle reflex, including eye-blink and post-auricular muscle response, were recorded in some human studies of GPIAS using electromyography or motion-tracking system [
28, 29, 30]. Recording EEG is another way to study gap detection ability and GPIAS in human studies. In this regard, three studies have recorded the mismatched negativity (MMN) using the gap deviation paradigm in tinnitus. In the other two human studies, CAEP has been used, one via the GIN stimulus pattern and the other by GPIAS.
Discussion
Continuous advances in the study of GPIAS in experimental tinnitus animal models have led to the emergence of different methods of GPIAS recording as objective research and diagnostic tool in tinnitus. Among the various methods, multi-channel CAEP recording is a new and compelling option as a biomarker of healthy brain circuitry. Cortical responses with the GPIAS paradigm investigate tinnitus’ cognitive, attentional, and temporal processing defects. The method of detecting tinnitus with GPIAS is based on the “filling the gap” theory. It hypotheses that when the gap embedded in the background noise holds the same frequency as the tinnitus, gap inhibition does not occur in the startle response because tinnitus “fills in” the silent gaps in the background noise. However, some studies have proven against it. When the background noise frequency opposes the tinnitus frequency, a startle inhibition defect is sometimes induced [
29, 30]. However, these studies do not rule out that the GPIAS indicates tinnitus. To further test the “filling the gap” hypothesis, human studies are preferable to animal studies because the perception of tinnitus can be addressed.
Many neurophysiological tinnitus models agree on maladaptive cortical and subcortical plasticity due to reduced auditory input (deafferentation) as a neural basis of tinnitus [
31-
33]. According to the edge theory, hyperactivity occurs in the adjacent regions with hearing loss [
34]. Numerous studies have demonstrated correlations between the predominant pitch of tinnitus and the edge frequency [
35, 36]. However, some inconsistent evidence exists [
37, 38]. Since the auditory cortex is one of the hubs involved in tinnitus networks (due to an increase in neuronal excitability or an increase in synchrony in the auditory cortex) [
39], changes in the dynamic properties of auditory cortex responses (spatial distribution, amplitude, and latency of CAEP components) can be utilized as objective diagnostic tools in tinnitus. Although some studies have found differences between the tinnitus and control groups in the early response of N1 and the late response of P300 [
40, 41], there is still no agreement on which component may be a biomarker of tinnitus [
42-
45]. The existent objective methods rely on differentiating tinnitus from non-tinnitus based on the morphology of subcortical/cortical responses [
46] and the pattern of EEG activity [
47]. The EEG and AEP methods with the machine learning algorithms, source analysis, and multiscale entropy measures have provided a new possibility to more accurately differentiate tinnitus neural activity from non-tinnitus and compare different subtypes of tinnitus [
48]. Source localization of CAEP is preferable rather than more complex machine learning methods to optimize the interpretability of the results [
49].
The auditory cortex is essential in temporal acuity. Gap detection is one way to measure temporal processing, both in humans and animals. Recording CAEPs with gap-embedded stimulus patterns is a new perspective to assess tinnitus. Although the brainstem circuit regulates the startle reflex, the auditory cortex contributes to the discovery gap detection in the GPIAS stimulus pattern [
19] due to the corticofugal radiations from the auditory cortex’s layer 5 and 6 neurons to the superior colliculus, inferior colliculus, olivary complex, cholinergic pedunculopontine tegmental area and the cochlear nucleus [
50]. According to the reviewing related articles, only two human studies recorded CAEP with gap stimuli. The GIN stimulus paradigm in Morse et al.’s study showed no significant differences in gap-evoked CAEPs between the tinnitus and control groups [
51]. However, Ku et al. recorded CAEPs with the GPIAS paradigm and observed the effect of tinnitus on the N1-P2 complex only in a 20-ms gap duration and 8 kHz background noise. The inhibition deficit was not marked in both groups, with a 50-ms gap duration and tinnitus pitch unmatched by the background noise frequency (600 Hz) [
52]. The results of this study do not support the concept of tinnitus filling in the gap and propose additional studies on the effects of background frequencies and gap duration on gap processing. The results of cortical inactivation studies in animals have shown that the auditory cortex is necessary to detect short gaps (<50 ms) but not for longer gaps (75-100 ms). Weible et al. study showed that cortical interneurons that constantly compare spike activity before and after the gap are involved in detecting gaps shorter than 25 ms [
53]. Cortex gap termination response neurons are neural manifestations of detecting short gaps [
54].
In a comprehensive review, the effectiveness of the GPIAS method was studied for the objective detection of tinnitus, but all human and animal studies with various response recording methods were studied. Consequently, the difference in stimulation parameters and data interpretation between laboratories was mentioned. Also, it was emphasized and recommended the preference for using human samples (due to the possibility of controlling the perception of tinnitus) and recording the evoked potentials with gap stimuli [
55]. In a 2020 review paper, studies on EEG evoked by gap-embedded stimuli to detect tinnitus were collected and reviewed [
56]. In that study, all brain responses with short, medium, and long latencies and all stimuli with gaps were reviewed. Still, the current review focuses on CAEP studies recorded with GPIAS stimuli in humans only. The difference between the current literature review and other previous review studies is the focus on the cortical evoked potential recording method (not other reflex-based methods or other evoked potentials), GPIAS stimuli (not other gap-embedded stimuli), and human samples suffering from tinnitus (not tinnitus induced animals). Although few studies were conducted to analyze these criteria, the possibility of applying cortical responses with GPIAS stimuli was soon optimistic, and it was recommended to conduct more extensive studies.
The results of the previous reviews indicate that it is difficult to interpret the effect of tinnitus on gap processing due to the small number of studies and different study methodologies. One of the reasons for these contradictions is the inherent variability of the acoustic startle reflex, which subsequently affects the efficacy of GPIAS in assessing tinnitus. Factors influencing this variability have been investigated in various studies on animal models, including startle stimulus and background noise properties (type, intensity, frequency), the distance between startle stimulus and gap (-stimulus interval), the distance between trials (inter-trial interval), gender differences, circadian rhythm, sample age (due to the effect of development and maturity), habituation, facilitation due to the gap, salience or importance of pre-pulse stimulus and attention to the pre-pulse stimulus (in humans) [
57, 58]. Determining the most effective parameters differentiating tinnitus from normal conditions in the GPIAS method leads to its validation and standardization for tinnitus diagnosis. Among the factors mentioned above, the factors of gap position, gap duration, gap distance from the startle, and background noise frequency are the most influential. Although longer gaps provide more inhibition in the startle response [
59,
60], only short gaps with distance from the startle (gap-embedded situation) process in the auditory cortex can be well evaluated with the CAEP tool [
54]. In most PPI studies, intervals of 30 to 240 are usually used between the gap and startle; however, the results of studies have shown that the maximum inhibition occurs at a distance of 100-120 ms [
22,
61]. The background noise frequency mainly affects the gap processing, and therefore in some studies, even normal people have shown less inhibition in low-frequency background noise [
52,
62]. Hence, it is recommended to accurately match high-frequency background noise with tinnitus frequency and use a frequency higher than 1 kHz as low-frequency background noise to reduce the inherent effect of frequency on gap processing [
52]. Further studies on this method are needed to determine if the defects of GPIAS are due to tinnitus or other abnormalities in the auditory or nervous system.
Conclusion
Although objective markers, such as imaging techniques or electrophysiological responses, have been studied to prove the presence or effects of tinnitus, no objective tool has been yet introduced to detect tinnitus in humans. There is no consensus among studies on the GPIAS method to assess tinnitus in humans. However, the possibility that the defects of GPIAS can be interpreted as an indicator of tinnitus has not been ruled out. To determine the efficacy of GPIAS in the proper and objective evaluation of tinnitus, human studies are the most logical because the perception of tinnitus can be controlled. An appealing research line in this area is EEG-based cortical evoked potentials. By manipulating acoustic stimulus parameters during CAEP recording, it is possible to determine the most influential parameter for the diagnosis of tinnitus and possibly explain the difference between the results of the studies.
Ethical Considerations
Compliance with ethical guidelines
The authors attest that all ethical considerations have been considered in compiling and referencing the content.
Funding
This research did not receive any grant from funding agencies in the public, commercial, or non-profit sectors.
Authors' contributions
Conceptualization and supervision: Akram Pourbakht; Methodology: Seyyed Jalal Sameni and Malihe Mazaheryazdi; Investigation and writing–review & editing: All authors; Writing–original draft: Soheila Shayanmehr; Funding acquisition and resources: Nariman Rahbar.
Conflict of interest
The authors declared no conflict of interest.
Acknowledgments
The authors would like to thank the department of audiology for cooperation and help to design the study.
References
- Bhatt JM, Lin HW, Bhattacharyya N. Prevalence, Severity, exposures and treatment patterns in the United States. JAMA Otolaryngology-- Head & Neck Surgery. 2016; 142(10):959-65. [DOI:10.1001/jamaoto.2016.1700.] [PMID] [PMCID]
- McCormack A, Edmondson-Jones M, Somerset S, Hall D. A systematic review of the reporting of tinnitus prevalence and severity. Hearing Research. 2016; 337:70-9. [DOI:10.1016/j.heares.2016.05.009] [PMID]
- Axelsson A, Ringdahl A. Tinnitus-a study of its prevalence and characteristics. British Journal of Audiology. 1989; 23(1):53-62.[DOI:10.3109/03005368909077819] [PMID]
- Lee DY, Lee JY, Kim YH. Management of tinnitus in children: Review of literature and effect of counseling. Auris, Nasus, Larynx. 2018; 45(4):667-72. [DOI:10.1016/j.anl.2017.09.002] [PMID]
- Hébert S, Canlon B, Hasson D, Magnusson Hanson LL, Westerlund H, Theorell T. Tinnitus severity is reduced with reduction of depressive mood - a prospective population study in Sweden. Plos One. 2012;7(5):e37733. [DOI:10.1371/journal.pone.0037733.] [PMID] [PMCID]
- De Ridder D, Schlee W, Vanneste S, Londero A, Weisz N, Kleinjung T, et al. Tinnitus and tinnitus disorder: Theoretical and operational definitions (an international multidisciplinary proposal). Progress in Brain Research. 2021; 260:1-25. [PMID]
- Tunkel DE, Bauer CA, Sun GH, Rosenfeld RM. American Academy of Otolaryngology—Head and Neck Foundation clinical practice guideline: Tinnitus. Otolaryngology—Head and Neck Surgery. 2014; 151(S1):20. [DOI:10.1177/0194599814538403a56]
- Ogawa K, Sato H, Takahashi M, Wada T, Naito Y, Kawase T, et al. Clinical practice guidelines for diagnosis and treatment of chronic tinnitus in Japan. Auris, Nasus, Larynx. 2020; 47(1):1-6. [DOI:10.1016/j.anl.2019.09.007] [PMID]
- Genitsaridi E, Hoare DJ, Kypraios T, Hall DA. A review and a framework of variables for defining and characterizing tinnitus subphenotypes. Brain Sciences. 2020; 10(12):938. [DOI:10.3390/brainsci10120938] [PMID] [PMCID]
- Swain SK, Nayak S, Ravan JR, Sahu MC. Tinnitus and its current treatmenteStill an enigma in medicine. Journal of the Formosan Medical Association. 2016; 115(3):139-44. [DOI:10.1016/j.jfma.2015.11.011] [PMID]
- De Ridder D, Vanneste S, Weisz N, Londero A, Schlee W, Elgoyhen AB, et al. An integrative model of auditory phantom perception: Tinnitus as a unified percept of interacting separable subnetworks.Neuroscience and Biobehavioral Reviews. 2014; 44:16-32. [DOI:10.1016/j.neubiorev.2013.03.021] [PMID]
- Boyen K, Başkent D, van Dijk P. The Gap Detection Test: Can It be used to diagnose tinnitus? Ear and Hearing. 2015; 36(4):138-45. [DOI:10.1097/AUD.0000000000000156] [PMID] [PMCID]
- Han BI, Lee HW, Kim TY, Lim JS, Shin KS. Tinnitus: Characteristics, causes, mechanisms, and treatments. Journal of Clinical Neurology. 2009; 5(1):11-9. [DOI:10.3988/jcn.2009.5.1.11] [PMID] [PMCID]
- JeanNoreña A. An integrative model of tinnitus based on a central gain controlling neural sensitivity. Neuroscience and Biobehavioral Reviews. 2011; 35(5):1089-109. [DOI:10.1016/j.neubiorev.2010.11.003] [PMID]
- Holgers k-M, Barrenas ML, Svedlund J, Zöger S. Clinical evaluation of tinnitus: a review. Audiological Medicine . 2009; 1(2):101-6. [DOI: doi.org/10.1080/16513860301714]
- Koops EA, Renken RJ, Lanting CP, van Dijk P. Cortical tonotopic map changes in humans are larger in hearing loss than in additional tinnitus. The Journal of Neuroscience. 2020; 40(16):3178-85. [DOI:10.1523/JNEUROSCI.2083-19.2020] [PMID] [PMCID]
- Persic D, Thomas ME, Pelekanos V, Ryugo DK, Takesian AE, Krumbholz K, et al. Regulation of auditory plasticity during critical periods and following hearing loss. Hearing Research. 2020; 397:107976. [DOI:10.1016/j.heares.2020.107976] [PMID] [PMCID]
- Fournier P, Hébert S. The gap-startle paradigm to assess auditory temporal processing: Bridging animal and human research. Psychophysiology. 2016; 53(5):759-66. [DOI:10.1111/psyp.12620] [PMID]
- Li L, Du Y, Li N, Wu X, Wu Y. Top-down modulation of prepulse inhibition of the startle reflex in humans and rats. Neuroscience and Biobehavioral Reviews. 2009; 33(8):1157-67. [DOI:10.1016/j.neubiorev.2009.02.001] [PMID]
- Yu H, Vikhe Patil K, Han C, Fabella B, Canlon B, Someya S, et al. GLAST deficiency in mice exacerbates gap detection deficits in a model of salicylate-induced tinnitus. Frontiers in Behavioral Neuroscience. 2016; 10:158. [DOI:10.3389/fnbeh.2016.00158] [PMID]
- Moreno-Paublete R, Canlon B, Cederroth CR. Differential neural responses underlying the inhibition of the startle response by pre-pulses or gaps in mice. Frontiers in Cellular Neuroscience. 2017; 11:19. [DOI:10.3389/fncel.2017.00019] [PMID] [PMCID]
- Braff DL, Geyer MA, Swerdlow NR. Human studies of prepulse inhibition of startle: Normal subjects, patient groups, and pharmacological studies. Psychopharmacology. 2001; 156(2-3):234-58.[DOI:10.1007/s002130100810] [PMID]
- Campbell LE, Hughes M, Budd TW, Cooper G, Fulham WR, Karayanidis F, et al. Primary and secondary neural networks of auditory prepulse inhibition: A functional magnetic resonance imaging study of sensorimotor gating of the human acoustic startle response. The European Journal of Neuroscience. 2007; 26(8):2327-33.[DOI:10.1111/j.1460-9568.2007.05858.x] [PMID]
- Kumari V, Antonova E, Geyer MA, Ffytche D, Williams SC, Sharma T. A fMRI investigation of startle gating deficits in schizophrenia patients treated with typical or atypical antipsychotics.The International Journal of Neuropsychopharmacology. 2007; 10(4):463-77. [DOI:10.1017/S1461145706007139] [PMID]
- Kumari V, Gray JA, Geyer MA, ffytche D, Soni W, Mitterschiffthaler MT, et al. Neural correlates of tactile prepulse inhibition: A functional MRI study in normal and schizophrenic subjects. Psychiatry Research. 2003; 122(2):99-113. [DOI:10.1016/S0925-4927(02)00123-3] [PMID]
- Rezapour M, Akbari M, Dargahi L, Zibaii MI, Shahbazi A. The Auditory Brainstem Response (ABR) test, supplementary to behavioral tests for evaluation of the salicylate-induced tinnitus. Indian Journal of Otolaryngology and Head and Neck Surgery. 2023; 75(Suppl 1):6-15. [DOI:10.1007/s12070-022-03117-x.] [PMID]
- Campolo J, Lobarinas E, Salvi R. Does tinnitus “fill in” the silent gaps? Noise & Health. 2013; 15(67):398-405. [DOI:10.4103/1463-741.121232.] [PMID] [PMCID]
- Fournier P, Hébert S. Gap detection deficits in humans with tinnitus as assessed with the acoustic startle paradigm: Does tinnitus fill in the gap? Hearing Research. 2013; 295:16-23. [DOI:10.1016/j.heares.2012.05.011] [PMID]
- Shadwick K, Sun W. Acoustic startle reflex and pre-pulse inhibition in tinnitus patients. Journal of Otology. 2014; 9(3):141-5. [DOI:10.1016/j.joto.2014.12.003]
- Wilson CA, Berger JI, de Boer J, Sereda M, Palmer AR, Hall DA, et al. Gap-induced inhibition of the post-auricular muscle response in humans and guinea pigs. Hearing Research. 2019; 374:13-23. [DOI:10.1016/j.heares.2019.01.009] [PMID] [PMCID]
- De Ridder D, Vanneste S, Langguth B, Llinas R. Thalamocortical dysrhythmia: A theoretical update in tinnitus. Frontiers in Neurology. 2015; 6:124. [DOI:10.3389/fneur.2015.00124.] [PMID] [PMCID]
- Vanneste S, De Ridder D. Deafferentation-based pathophysiological differences in phantom sound: Tinnitus with and without hearing loss. Neuroimage. 2016; 129:80-94. [DOI:10.1016/j.neuroimage.2015.12.002] [PMID]
- Eggermont JJ. Central tinnitus. Auris, Nasus, Larynx 2003; 30 Suppl:S7-12. [DOI:10.1016/S0385-8146(02)00122-0] [PMID]
- Noreña AJ, Farley BJ. Tinnitus-related neural activity: Theories of generation, propagation, and centralization. Hearing Research. 2013; 295:161-71. [DOI:10.1016/j.heares.2012.09.010] [PMID]
- Moore BC, Vinay, Sandhya. The relationship between tinnitus pitch and the edge frequency of the audiogram in individuals with hearing impairment and tonal tinnitus. Hearing Research. 2010; 261(1-2):51-6. [DOI:10.1016/j.heares.2010.01.003] [PMID]
- Savastano M. Tinnitus with or without hearing loss: Are its characteristics different? European Archives of Oto-Rhino-Laryngology. 2008; 265(11):1295-300. [DOI:10.1007/s00405-008-0630-z] [PMID]
- Schecklmann M, Vielsmeier V, Steffens T, Landgrebe M, Langguth B, Kleinjung T. Relationship between Audiometric Slope and tinnitus pitch in tinnitus patients: Insights into the mechanisms of tinnitus generation. Plos One. 2012; 7(4):e34878. [PMID] [PMCID]
- Flores LS, Teixeira AR, Rosito LP, Seimetz BM, Dall'Igna C. Pitch and loudness from tinnitus in individuals with noise-induced hearing loss. International Archives of Otorhinolaryngology. 2016; 20(3):248-53. [DOI:10.1055/s-0035-1562935] [PMID] [PMCID]
- Houdayer E, Teggi R, Velikova S, Gonzalez-Rosa JJ, Bussi M, Comi G, et al. Involvement of cortico-subcortical circuits in normoacousic chronic tinnitus: A source localization EEG study. Clinical Neurophysiology. 2015; 126(12):2356-65. [DOI:10.1016/j.clinph.2015.01.027] [PMID]
- Gopal KV, Thomas BP, Nandy R, Mao D, Lu H. Potential audiological and MRI markers of tinnitus. Journal of the American Academy of Audiology. 2017; 28(8):742-57. [DOI:10.3766/jaaa.16106] [PMID]
- Santos Filha VA, Matas CG. Late Auditory evoked potentials in individuals with tinnitus. Brazilian Journal of Otorhinolaryngology. 2010; 76(2):263-70. [DOI:10.1590/S1808-86942010000200019] [PMID] [PMCID]
- Gabr TA, El-Hay MA, Badawy A. Electrophysiological and psychological studies in tinnitus. Auris, Nasus, Larynx. 2011; 38(6):678-83. [DOI:10.1016/j.anl.2011.02.001] [PMID]
- Cardon E, Joossen I, Vermeersch H, Jacquemin L, Mertens G, Vanderveken OM, et al. Systematic review and meta-analysis of late auditory evoked potentials as a candidate biomarker in the assessment of tinnitus. Plos One. 2020; 15(12):e0243785. [PMID] [PMCID]
- Seraji H, Mohammadkhani G, Taghavi SMR. Applications of auditory evoked potentials in tinnitus: A review. Auditory and Vestibular Research Journal. 2021; 30(4):220-31. [DOI:10.18502/avr.v30i4.7443]
- Jackson R, Vijendren A, Phillips J. Objective measures of tinnitus: A systematic review. Otology & Neurotology. 2019; 40(2):154-63. [DOI:10.1097/MAO.0000000000002116] [PMID]
- Liu Y, Niu H, Zhu J, Zhao P, Yin H, Ding H, et al. Morphological neuroimaging biomarkers for tinnitus: Evidence obtained by applying machine learning. Neural Plasticity. 2019; 2019:1712342. [PMID] [PMCID]
- Vanneste S, Song JJ, De Ridder D. Thalamocortical dysrhythmia detected by machine learning. Nature Communications. 2018; 9(1):1103. [DOI:10.1038/s41467-018-02820-0] [PMID] [PMCID]
- Sadeghijam M, Talebian S, Mohsen S, Akbari M, Pourbakht A. Shannon entropy measures for EEG signals in tinnitus. Neuroscience Letters. 2021; 762:136153. [DOI:10.1016/j.neulet.2021.136153] [PMID]
- Cardon E, Vermeersch H, Joossen I, Jacquemin L, Mertens G, Vanderveken OM, et al. Cortical auditory evoked potentials, brain signal variability and cognition as biomarkers to detect the presence of chronic tinnitus. Hearing Research. 2022; 420:108489. [PMID]
- Doucet JR, Molavi DL, Ryugo DK. The source of corticocollicular and corticobulbar projections in area Te1 of the rat. Experimental Brain Research. 2003; 153(4):461-6. [DOI:10.1007/s00221-003-1604-4] [PMID]
- Morse K, Vander Werff KR. Comparison of silent gap in noise cortical auditory evoked potentials in matched tinnitus and no-tinnitus control subjects. American Journal of Audiology. 2019; 28(2):260-73.[DOI:10.1044/2018_AJA-18-0074] [PMID]
- Ku Y, Ahn JW, Kwon C, Kim DY, Suh MW, Park MK, et al. The gap-prepulse inhibition deficit of the cortical N1-P2 complex in patients with tinnitus: The effect of gap duration. Hearing Research. 2017; 348:120-8. [PMID]
- Weible AP, Moore AK, Liu C, DeBlander L, Wu H, Kentros C, et al. Perceptual gap detection is mediated by gap termination responses in auditory cortex. Current Biology. 2014; 24(13):1447-55. [DOI:10.1016/j.cub.2014.05.031] [PMID] [PMCID]
- Threlkeld SW, Penley SC, Rosen GD, Fitch RH. Detection of silent gaps in white noise following cortical deactivation in rats. Neuroreport. 2008; 19(8):893-8. [DOI:10.1097/WNR.0b013e3283013d7e] [PMID] [PMCID]
- Galazyuk A, Hebert S. Gap-prepulse inhibition of the acoustic startle reflex (GPIAS) for tinnitus assessment: Current status and future directions. Frontiers in Neurology. 2015; 6:88. [DOI:10.3389/fneur.2015.00088] [PMID] [PMCID]
- Duda V, Scully O, Baillargeon MS, Hébert S. Does tinnitus fill in the gap using electrophysiology? A scoping review. Otolaryngologic Clinics of North America. 2020; 53(4):563-82. [DOI:10.1016/j.otc.2020.03.006] [PMID]
- Longenecker RJ, Galazyuk AV. Methodological optimization of tinnitus assessment using prepulse inhibition of the acoustic startle reflex. Brain Research. 2012; 1485:54-62. [DOI:10.1016/j.brainres.2012.02.067] [PMID]
- Longenecker RJ, Kristaponyte I, Nelson GL, Young JW, Galazyuk AV. Addressing variability in the acoustic startle reflex for accurate gap detection assessment. Hearing Research. 2018; 363:119-35. [DOI:10.1016/j.heares.2018.03.013] [PMID] [PMCID]
- Barsz K, Ison JR, Snell KB, Walton JP. Behavioral and neural measures of auditory temporal acuity in aging humans and mice. Neurobiology of Aging. 2002; 23(4):565-78. [DOI:10.1016/S0197-4580(02)00008-8] [PMID]
- Allen PD, Schmuck N, Ison JR, Walton JP. Kv1.1 channel subunits are not necessary for high temporal acuity in behavioral and electrophysiological gap detection.Hearing Research. 2008; 246(1-2):52-8. [DOI:10.1016/j.heares.2008.09.009] [PMID] [PMCID]
- Oranje B, Geyer MA, Bocker KB, Leon Kenemans J, Verbaten MN. Prepulse inhibition and P50 suppression: Commonalities and dissociations. Psychiatry Research. 2006; 143(2-3):147-58.[DOI:10.1016/j.psychres.2005.11.002] [PMID]
- Atcherson SR, Gould HJ, Mendel MI, Ethington CA. Auditory N1 component to gaps in continuous narrowband noises. Ear and Hearing. 2009; 30(6):687-95. [DOI:10.1097/AUD.0b013e3181b1354f] [PMID]
- Mahmoudian S, Farhadi M, Najafi-Koopaie M, Darestani-Farahani E, Mohebbi M, Dengler R, et al. Central auditory processing during chronic tinnitus as indexed by topographical maps of the mismatch negativity obtained with the multi-feature paradigm. Brain Research. 2013; 1527:161-73. [DOI:10.1016/j.brainres.2013.06.019] [PMID]
- Mehdizade Gilani V, Ruzbahani M, Mahdi P, Amali A. Temporalprocessing evaluation in tinnitus patients: Results on analysis of gap in noise and duration pattern test. Iranian Journal of Otorhinolaryngology. 2013; 25(4):221-5. [Link]
- Mahmoudian S, Farhadi M, Mohebbi M, Alaeddini F, Najafi-Koopaie M, Farahani ED, et al. Alterations in auditory change detection associated with tinnitus residual inhibition induced by auditory electrical stimulation. Journal of the American Academy of Audiology. 2015; 26(4):408-22. [DOI:10.3766/jaaa.26.4.8] [PMID]
- Mohebbi M, Daneshi A, Asadpour A, Mohsen S, Farhadi M, Mahmoudian S. The potential role of auditory prediction error in decompensated tinnitus: An auditory mismatch negativity study. Brain Behavior. 2019; 9(4):e01242. [PMID]